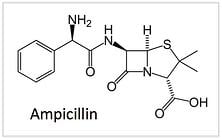
What are antibiotics?
Antibiotics are generally defined as agents that kill bacteria, or inhibit their growth. Although originally sourced from natural products, many common antibiotics used in labs today are semi-synthetic or fully synthetic compounds. Antibiotics can be categorized based on whether they directly kill bacteria (bactericidal) or slow growth/prevent cell division (bacteriostatic); however, the distinction between the two categories may be a bit of a gray area as some bacteriostatic reagents can kill bacteria when used at high concentrations (and vice versa). Looking around the lab, you'll likely find many of the antibiotics listed in the table below. Note, in this post we'll focus primarily on antibiotics against Gram negative bacteria. In future posts, we'll detail selection in non-bacterial cells such as yeast or mammalian cells.
Name | Class | Mode of Action* | Working Concentration** | |
---|---|---|---|---|
Kanamycin |
aminoglycoside |
Binds 30S ribosomal subunit; causes mis-translation | Bactericidal | 50-100 ug/mL |
Spectinomycin | aminoglycoside |
Binds 30S ribosomal subunit; interrupts protein synthesis |
Bactericidal |
7.5-50 ug/mL |
Streptomycin | aminoglycoside |
Inhibits initiation of protein synthesis |
Bactericidal |
25-100 ug/mL |
Ampicillin |
beta-lactam |
Inhibits cell wall synthesis | Bactericidal | 100-200 ug/mL |
Carbenicillin |
beta-lactam |
Inhibits cell wall synthesis | Bactericidal | 100 ug/mL |
Bleomycin |
glycopeptide |
Induces DNA breaks | Bactericidal | 5-100 ug/mL |
Erythromycin | macrolide |
Blocks 50S ribosomal subunit; inhibits aminoacyl translocation |
Bacteriostatic |
50-100 ug/mL in EtOH |
Polymyxin B |
polypeptide |
Alters outer membrane permeability | Bactericidal | 10-100 ug/mL |
Tetracycline | tetracyclin |
Binds 30S ribosomal subunit; inhibits protein synthesis (elongation step) |
Bacteriostatic |
10 ug/mL |
Chloramphenicol | Binds 50S ribosomal subunit; inhibits peptidyl translocation | Bacteriostatic | 5-25 ug/mL in EtOH |
*In prokaryotes. **Dissolve in dH2O and sterile filter unless otherwise specified.
The above table lists some antibiotics commonly found in the lab, their mechanism for killing bacteria, and general working concentrations. For instructions on how to prepare antibiotic stocks, see Addgene's Reference Page.
How else can antibiotics be used in the lab?
Historically, antibiotics have also been used to disrupt genes at the chromosomal level. Scientists introduce an antibiotic resistance cassette within the coding region of the gene they are trying to disrupt or delete, which both inactivates the gene and acts as a marker for the mutation. When designing these types of experiments it is best practice not to use the same resistance cassette for the mutation and for plasmid selection. Additionally, scientists can use the loss of resistance as a marker for successful cloning. In these instances, the cloning vector typically has two separate resistance cassettes and your gene of interest is cloned into/inactivates or completely removes (in the case of Gateway cloning) one cassette. Counterselection allows the scientist to select bacteria that are only resistant to the antibiotic that remains intact.
Tips and tricks from the bench
- Use fresh stocks. Most antibiotics are stable in powder form, but quickly breakdown in solution. Storing aliquots at -20oC and avoiding repeated freeze/thaw cycles will keep most antibiotics viable for at least 6 months.
- Ampicillin breaks down especially fast and plates should be used witin 1 month for optimal efficiency. Beware of satellite colonies!
- Carbenicillin is more stable than Ampicillin and can be used in place of Ampicillin in most applications.
- Antibiotics vary in their sensitivitly to heat and/or light – do not add them to media hotter than about 55oC and store plates/stocks wrapped in foil if a light-sensitive antibiotic like Tetracycline is used.
- Keep in mind that some E. coli strains have natural antibiotic resistances, so make sure your plasmid and E. coli strain are compatible! Check out this list of common E. coli genotypes and their natural resistances.
Additional Resources on the Addgene Blog
- Read other Plasmids 101 Posts
- Learn How to Use Blue White Screening to Simplify Your Cloning Experiments
- See Where You Can Find Antibiotic Resistance on Our Plasmid Pages
Resources on Addgene.org
- Browse Our Molecular Biology Reference Pages
- View our Plasmid Protocols
- Find Your Perfect Plasmid Backbone
Topics: Plasmid Elements, Plasmids 101, Plasmids
Leave a Comment