Originally published Oct 11, 2016 and last updated Dec 22, 2020 by Benoit Giquel.
CRISPR has taken the genome engineering world by storm owing to its ease of use and utility in a wide variety of organisms. While much of current CRISPR research focuses on its potential applications for human medicine (Waltz, 2016), the potential of CRISPR for genome engineering in plants is also being realized. There are a variety of reasons to consider using genome editing to change the genetic code of plants, including the development of crops with longer shelf life and the development of disease-resistant crops to increase agricultural yield (Wang et al., 2016; Wang et al., 2014). While it is certainly possible to select for desirable traits using traditional plant breeding approaches, these techniques are cumbersome, often requiring several rounds of selection to isolate plants with the phenotype of interest. Genome engineering, on the other hand, allows for targeted modification of known or suspected genes that regulate a desired phenotype. In fact, CRISPR has already been used to engineer the genome of many plant species, including commonly used model organisms like Arabidopsis and Medicago truncatula and several crop species including potato, corn, tomato, wheat, mushroom, and rice (Khatodia et al., 2016). Despite the almost universal functionality of the CRISPR system in most organisms, some plant-specific changes to CRISPR components are necessary to enable genome editing in plant cells.
This blog post will present a general overview of plant genome engineering using CRISPR, highlight the specific modifications to CRISPR machinery that allow for the use of CRISPR in plants, and outline the various plant genome engineering tools that are available to academic researchers through Addgene.
CRISPR components for plant genome engineering
CRISPR can be used to knockout, activate or repress target genes in plants using the same general experimental design principles developed in other model organisms (see our CRISPR guide for common CRISPR principles). However, plant-specific modifications to commonly used CRISPR plasmids are necessary to use the CRISPR system in plant cells. Like other model systems, expression of S. pyogenes Cas9 or Cas9 variants (hereafter referred to as Cas9) and a single stranded guide RNA (gRNA) is sufficient to modify the genome of plant cells. The structure of the gRNA (composed of a ~20 nucleotide targeting sequence and ~75 nucleotide scaffold sequence) is consistent between plants and other organisms, but the promoter used to drive gRNA expression is dependent upon the cell type in question. In plant cells, gRNA expression is achieved by placing the gRNA downstream of a plant-specific RNA pol III promoter, such as AtU6, TaU6, OsU6 or OsU3, which are commonly used to drive expression of small RNAs in their respective species. Addgene carries >30 “empty gRNA” backbones which contain a plant pol III promoter and gRNA scaffold sequence and allow researchers to insert targeting oligos with minimal cloning required. As with other model systems, multiple gRNAs can be expressed to modify several genomic loci at once (get more information on multiplexing gRNAs here).
Cas9 is commonly tagged with a nuclear localization sequence to enhance targeting to the nucleus, and several codon optimized Cas9 variants have been created in an effort to increase translation in a particular plant species or cell type (Belhaj et al., 2013). Nuclease dead Cas9 (dCas9) based activators (such as dCas9-VP64) or repressors (dCas9-KRAB or dCas9-SRDX) can also be used to activate or repress target genes in plant cells, respectively. Cas9 expression is commonly driven by plant-derived RNA pol II promoters which regulate expression of longer RNAs (such as mRNAs for gene expression). Examples of commonly used RNA pol II promoters for Cas9 expression include the ubiquitously expressing cauliflower mosaic virus 35S promoter (CaMV 35S) or ubiquitin promoters (Belhaj et al., 2013). Addgene carries Cas9-containing plasmids for knockout, activation and repression of target genes in plants and many of the aforementioned empty gRNA backbones also contain Cas9, which enables expression of both Cas9 and the gRNA off of the same plasmid.
Delivering CRISPR components to plant cells
Once you have selected the correct CRISPR components for your application, it is time to deliver these components to your target cells. Remember, efficient delivery of CRISPR components is essential for any CRISPR experiment, and failure to express either the gRNA or Cas9 in your cell line will result in a failed experiment. CRISPR components can be expressed stably or transiently depending on the delivery method and cell type in question. CRISPR components can be delivered and expressed transiently using a standard detergent, Polyethylene Glycol (PEG), although the application of this approach is limited to protoplast cells (plant cells whose cell wall has been removed). Another common delivery method is agrobacterium-mediated delivery, which uses the soil derived bacterium Agrobacterium tumefaciens as a vehicle to deliver your gene of interest into a target cell line or organism (Presented in figure 1). More information on Agrobacterium-mediated transformation can be found in this blog post. The pDGE Dicot Genome Editing Kit from the Stuttmann lab contains a variety of Agrobacterium-compatible, Cas9 containing vectors ready for Golden Gate mediated cloning of your gRNA of interest.
In recent years, deaminase-mediated base editing (cytosine base editor or adenine base editor) and reverse transcriptase-mediated prime editing technologies have been shown to be excellent alternative genome editing technologies especially in human cells. In contrast to homology directed repair, these methods do not involve double strand break (DSB) formation and do not require donor DNA, . These precise edits tend to be more efficient than HDR (reviewed in Zhu et al., 2019). Both cytosine base editors and adenine base editors have been developed for plants and prime editing for plants has been developed for rice by Yiping Qi’s lab and for rice and wheat by Caixia Gao’s lab.
More recently developed methods can also be used to efficiently deliver CRISPR-Cas9 components into plants. Nanoparticles (carbon nanotubes, Kwak et al., 2019), DNA nanostructures (Zhang et al., 2019) or cell-penetrating peptides (Santana et al., 2020) and plants viruses (barley yellow striate mosaic virus (Gao et al., 2019) or sonchus yellow net rhabdovirus (Ma et al., 2020) have already been shown to be efficient ways to deliver CRISPR-Cas9 to plant cells and should be considered alternative to PEG or agrobacterium-mediated delivery.
Summary
While different in many of the specifics - promoters used, precise protein sequences or domains, and methods of delivery - the underlying technique of CRISPR mediated genome engineering in plants isn’t all that different from how it’s used in other systems. Luckily, you don’t have to look far for plasmids that have the plant-specific modifications required for targeting your favorite plant gene; you can find many plasmids for a wide variety of CRISPR applications in plants available through Addgene. In addition to the plasmids described above, Addgene carries several useful CRISPR toolkits for creating plant expression plasmids, including plant CRISPR plasmids from the Yiping Qi lab and the MoClo Plant Parts Kit from the Patron Lab. As with all plasmids in the repository, we highly recommend reading the associated publications or protocols to get the most out of the plasmid you chose for your experiment, but, if you’re working with plants, don’t be afraid to try your hand at CRISPR.
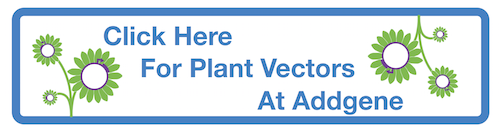
References
Belhaj K, Chaparro-Garcia A, Kamoun S, Nekrasov V (2013) Plant genome editing made easy: targeted mutagenesis in model and crop plants using the CRISPR/Cas system. Plant Methods 9:39 . https://doi.org/10.1186/1746-4811-9-39
Gao Q, Xu W, Yan T, Fang X, Cao Q, Zhang Z, Ding Z, Wang Y, Wang X (2019) Rescue of a plant cytorhabdovirus as versatile expression platforms for planthopper and cereal genomic studies. New Phytol 223:2120–2133 . https://doi.org/10.1111/nph.15889
Gelvin SB (2003) Agrobacterium-Mediated Plant Transformation: the Biology behind the “Gene-Jockeying” Tool. MMBR 67:16–37 . https://doi.org/10.1128/mmbr.67.1.16-37.2003
Khatodia S, Bhatotia K, Passricha N, Khurana SMP, Tuteja N (2016) The CRISPR/Cas Genome-Editing Tool: Application in Improvement of Crops. Front Plant Sci 7: . https://doi.org/10.3389/fpls.2016.00506
Kwak S-Y, Lew TTS, Sweeney CJ, Koman VB, Wong MH, Bohmert-Tatarev K, Snell KD, Seo JS, Chua N-H, Strano MS (2019) Chloroplast-selective gene delivery and expression in planta using chitosan-complexed single-walled carbon nanotube carriers. Nat Nanotechnol 14:447–455 . https://doi.org/10.1038/s41565-019-0375-4
Lowder LG, Zhang D, Baltes NJ, Paul JW III, Tang X, Zheng X, Voytas DF, Hsieh T-F, Zhang Y, Qi Y (2015) A CRISPR/Cas9 Toolbox for Multiplexed Plant Genome Editing and Transcriptional Regulation. Plant Physiol 169:971–985 . https://doi.org/10.1104/pp.15.00636
Ma X, Zhang X, Liu H, Li Z (2020) Highly efficient DNA-free plant genome editing using virally delivered CRISPR–Cas9. Nat Plants 6:773–779 . https://doi.org/10.1038/s41477-020-0704-5
Santana I, Wu H, Hu P, Giraldo JP (2020) Targeted delivery of nanomaterials with chemical cargoes in plants enabled by a biorecognition motif. Nat Commun 11: . https://doi.org/10.1038/s41467-020-15731-w
Wang F, Wang C, Liu P, Lei C, Hao W, Gao Y, Liu Y-G, Zhao K (2016) Enhanced Rice Blast Resistance by CRISPR/Cas9-Targeted Mutagenesis of the ERF Transcription Factor Gene OsERF922. PLoS ONE 11:e0154027 . https://doi.org/10.1371/journal.pone.0154027
Wang Y, Cheng X, Shan Q, Zhang Y, Liu J, Gao C, Qiu J-L (2014) Simultaneous editing of three homoeoalleles in hexaploid bread wheat confers heritable resistance to powdery mildew. Nat Biotechnol 32:947–951 . https://doi.org/10.1038/nbt.2969
Waltz E (2016) Gene-edited CRISPR mushroom escapes US regulation. Nature 532:293–293 . https://doi.org/10.1038/nature.2016.19754
Zhang H, Demirer GS, Zhang H, Ye T, Goh NS, Aditham AJ, Cunningham FJ, Fan C, Landry MP (2019) DNA nanostructures coordinate gene silencing in mature plants. Proc Natl Acad Sci USA 116:7543–7548 . https://doi.org/10.1073/pnas.1818290116
Zhu H, Li C, Gao C (2020) Applications of CRISPR–Cas in agriculture and plant biotechnology. Nat Rev Mol Cell Biol 21:661–677 . https://doi.org/10.1038/s41580-020-00288-9
Additional Resources on the Addgene Blog
Additional Resources on Addgene.org
- Find CRISPR Plasmids for Plants
- Read Our CRISPR Guide Pages
- Find Validated gRNAs for Your Next Experiment
Topics: CRISPR, Plant Biology, CRISPR 101
Leave a Comment