Bioluminescence and fluorescence from proteins such as Green Fluorescent Protein (GFP) has likely existed in creatures such as jellyfish for millions of years; however, it took until the 1960s for scientists to begin to study GFP and deduce its biochemical properties. Now GFP and its fluorescent derivatives are a staple in the lab. GFP is used in research across a vast array of biological disciplines and scientists employ GFP for a wide number of functions, including: tagging genes for elucidating their expression or localization profiles, acting as a biosensor or cell marker, studying protein-protein interactions, visualizing promoter activity, and much more.
Read on to learn more about GFP, how scientists have evolved this versatile protein to suit their experimental needs, and some of the common applications in the lab.

Why Green Fluorescent Protein?
GFP is a ~27 kDa protein consisting of 238 amino acids derived from the crystal jellyfish Aequorea victoria. It has a fluorescent emission wavelength in the green portion of the visible spectrum (hence the name), which is due to a chromophore formed from a maturation reaction of three specific amino acids at the center of the protein (Ser65, Tyr66, and Gly67). When first discovered, one of the most surprising aspects of GFP was the fact that the chromophore forms spontaneously and without additional co-factors, substrates, or enzymatic activity – it only requires the presence of oxygen during maturation. This meant that the protein could be taken directly from A. Victoria and expressed in any organism while still maintaining fluorescence.
The protein structure, first reported in 1996, is an eleven β-sheet-containing “barrel” shape, with the chromophore concealed at the center of the structure, shielded from quenching by aqueous solvent. This tightly-packed structure explains the importance of the entire GFP protein, which is almost completely required to maintain fluorescent activity; very little truncation is tolerated, however, point mutations are acceptable. GFP's main advantage over conventional fluorescent dyes of the time was the fact that it was non-toxic and could be expressed in living cells, enabling the study of dynamic, physiological processes.
Re-engineering GFP to increase its range of color and application
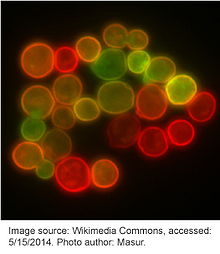
Almost as soon as its sequence was elucidated, scientists began engineering new versions of GFP through mutagenesis in order to improve its physical and biochemical properties. In 1995, Roger Y. Tsien described an S65T point mutation that increased the fluorescence intensity and photostability of GFP. This also shifted its major excitation peak from 395 nm to 488 nm, effectively ameliorating the deficiencies found in the wildtype protein and facilitating its widespread use in research. Many other mutations have since been introduced to GFP and new iterations of fluorophores are constantly being engineered. Table 1 below lists a few common fluorescent proteins and their mutations relative to wildtype GFP. Although not listed here, many permeations within each color also exist with only slight variations separating them.
Please note that many fluorescent proteins found on the red side of the spectrum are not GFP derivatives, but are instead related to the dsRed protein isolated from Discosoma sp. Similar work has been done to expand the red-fluorescent protein repertoire; however, these proteins are unique from GFP and the mutation definitions found in Table 2 may not apply.
Table 1: The specific mutations comprising common fluorophores
Fluorescent Protein | Mutations Relative to Wildtype GFP |
EGFP | F64L; S65T |
EYFP | S65G; V68L; S72A; T203Y |
mYFP | S65G; V68L; Q69K; S72A; T203Y; A206K |
Citrine | S65G; V68L; Q69K; S72A; T203Y |
ECFP | F64L; S65T, Y66W; N146I; M153T; V163A |
mCFP | F64L; S65T, Y66W; N146I; M153T; V163A; A206K |
Cerulean | F64L, S65T, Y66W, S72A, Y145A, H148D, N149I, M153T, V163A |
EBFP | F64L, S65T, Y66H, Y145F |
Table 2: Functional role of specific mutations in GFP derivatives
Mutation | Known Function |
S65T | Increased fluorescence, photostability, and a shift of the major excitation peak to 488 nm |
F64L | Increased folding efficiency at 37C |
Y66W | Causes the chromophore to form with an indole rather than phenol component (cyan derivatives) |
Y66H | Blue-shifts the wavelength (blue derivatives) |
Y145F | Increases quantum yield for BFP |
Y145A and H148D | Stabilizes the structure of Cerulean derivatives |
F99S, M153T, V163A | Improves folding at 37C, reduces aggregation at high concentrations |
T203Y | Red-shifts the wavelength (yellow derivatives) |
A206K | Interferes with dimer interface (monomeric derivatives) |
K26R, Q80R, N146H, H231L, (and probably others) | Neutral mutations |
A multitude of applications
Due to its size and ease of use, GFP and other fluorescent proteins have become a mainstay in molecular biology. Scientists can easily utilize GFP-containing plasmids as a means to many functional ends. We’ve listed our favorites below, but many other uses currently exist, and new GFP technology is constantly being developed!
- Fusion tagging: One of the most common uses, GFP can be fused to the N- or C-terminus of a protein, which allows the scientist to visualize when and where the gene is expressed. Click here to view Addgene’s collection of empty backbones for constructing fluorescent fusions.
- Transcription Reporter: Placing GFP under the control of a promoter of interest can be used to effectively monitor gene expression from that promoter in a given cell type. This type of transcription reporting was among the earliest uses of GFP.
- Förster resonance energy transfer (FRET): This is used to study the interactions between two proteins or between two domains of a protein that undergoes conformational change. Typcially two fluorescent proteins with overlapping excitation/emission spectra are used; one fused to each protein or domain being tested. Find FRET plasmids here.
- Split EGFP: An alternative to FRET, split EGFP has also been used to study protein-protein interactions. In this case, two portions of EGFP are fused to the proteins of interest, and when they come into close proximity, the two halves of EGFP undergo folding, maturation, and fluorescence.
- Biosensors: A wide array of GFP-based fluorescent biosensors has been designed to detect a variety of intracellular conditions, including ion (such as Ca2+) concentrations and pH, using a range of strategies such as FRET, calmodulin, and others. Review Addgene's collection of fluorescent biosensors here.
- Optogenetics: Scientists can use light to detect, measure, and control molecular signals, cells, and groups of cells in order to understand their activity and visualize the effects of alterations to this activity. Learn more about optogenetics at OpenOptogenetics and find optogeneic actuators and sensors at Addgene.
- Cell marking/selection: Expression constructs like plasmids often include GFP as a marker to help identify which cells have successfully taken up the plasmid. This can serve as an alternative to antibiotic selection. Plasmids of this type may have the GFP under the control of an additional promoter from that of the gene of interest, or expressed from the same transcript as the gene of interest, but after an internal ribosome entry site (IRES). This is oftentimes used in conjuction with FACS (see below).
- Fluorescence-activated cell sorting (FACS): This is a type of flow cytometry that separates mixtures of cells into distinct populations based on fluorescent signal. Thus, FACS can be used to separate cells expressing GFP from cells that are not.
- Developmental/transgenic uses: Because of its stability, GFP can be used in lineage tracking capacities in cell fate studies. It can also be used, when put under control of promoters of interest, to visualize the developmental stage at which these promoters are active. Further, GFP can label transgenically modified ES cells, which can then be used for implantation and generation of transgenic mice.
- Purification: GFP can be used as a general epitope tag for protein purification and a number of commercial antibodies to GFP are available.
- Others: We’ve really just scratched the surface of the potential applications for GFP. It has also been used to identify particular cell populations in drug screens, to visualize micrometastases in nude mice in cancer studies, act as a reporter for DNA double strand break repair, and to label pathogenic intracellular microbes to visualize host/pathogen interactions.
Do you have a favorite way to use GFP? Comment and tell us how!
And come back next week when we'll be featuring a guest blog post from Gal Haimovich of greenfluorescentblog. Gal will share his list of 10 things to keep in mind when choosing the best fluorescent protein to use in your upcoming experiments. In the meantime, if you haven't checked out Gal's blog, you should! He's got great articles and insight on the newest tools and techniques in fluorescent microscopy.
Read More:
Note: A. Max Juchheim contributed to the writing of this article.
Leave a Comment