The first time I heard about FRET during a journal club, my guitarist brain automatically thought about the raised element found on the neck of my guitar...not really useful for a biologist you would say. The student was of course talking about the now well-known FRET, aka Fluorescence (Förster) Resonance Energy Transfer, technique which allows the detection of molecules' interactions, modifications or dissociations in situ. Used since the mid-90s, this technique has revolutionised the way we apprehend molecular complexes and is still a very useful tool.
Like a guitar hero (that I’m not), FRET loves playing “live.” Indeed, FRET was one of the first techniques which enabled the measurement of single molecule interactions in living cells using a microscope. Historically, molecular interactions were detected by indirect means often using probes with the potential to target several molecules. By analogy, it was like pointing out a group of students in a university hall but not knowing if these students know or interact with each other. FRET reduced the scale of our perception about molecular interactions.
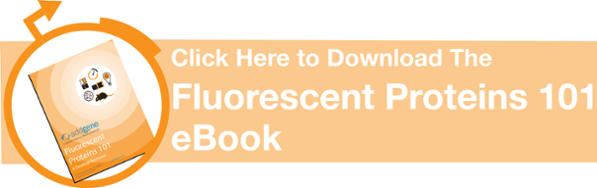
What is FRET?
In their JCB 2003 paper, Sekar and Periasamy defined FRET as "a distance-dependent physical process by which energy is transferred non-radiatively from an excited molecular fluorophore (the donor) to another fluorophore (the acceptor) by means of intermolecular long-range dipole-dipole coupling." The emission of the acceptor fluorophore can be measured using microscopy techniques. FRET measurement sensitivity makes it suitable for studying interactions within living cells. By coupling fluorophores to proteins, pioneers of this technique were able to directly detect protein/protein interactions in living cells. Since then FRET has also been used to measure conformational changes, cleavage activity using FRET-based biosensors, and interactions between DNA and proteins. FRET, in theory, is an easy technique, but it is very important to follow some simple rules to avoid common pitfalls and to use it at its best.
What are the Parameters that Affect FRET?
FRET occurs when the two fluorophores used are in close vicinity. Thus, the distance between the two fluorophores and their orientation to one another can affect FRET. When it comes to studying an unknown interaction between two proteins, these parameters are difficult to overcome but they have to be considered when analysing data from your FRET experiments. You may have to design several different constructs to find one that could be used for the purpose of your studies. These parameters are less important when designing a biosensor, as the distance between the two fluorophores and their spatial orientation will be fixed.
The quantum yield of the donor (number of emitted photons per absorbed photons) and the extinction coefficient of the acceptor (linking the quantity of absorbed light, at a given wavelength, to the concentration of fluorophore in solution) are two additional parameters that can affect FRET efficiency. These issues can be overcome by choosing a complementary pair of fluorophores. To maximize the FRET signal you should choose the highest quantum yield donor, the highest absorbing acceptor and fluorophores with significant overlap in their spectra. The pair CFP-YFP was the first to be used to study protein-protein interactions and several other pairs have been used since - including: mCerulean/mVenus, mCerulean/Amber, mCerulean/SYFP2A, mTurquoise/mVenus and others. CFP-YFP is still one of the best and most used pairs to measure FRET.
The table below lists plasmids that can be used to create your choice of fluorescent fusion protein with your gene of interest:
Expresses Aquamarine with N-terminal His tag | |||
Expresses mammalian optimized Aquamarine | |||
Express a gene of interest fused to the N-terminus of monomeric Cerulean | |||
Express a gene of interest fused to the C-terminus of monomeric Cerulean | |||
Constructs to target mTurquoise2 to various subcellular compartments | |||
Expresses mammalian optimized CyPet | |||
Expresses CyPet with C-terminal His tag | |||
Express a gene of interest fused to the C-terminus of SCFP3A | |||
Express a gene of interest fused to the N-terminus of Amber | |||
Express a gene of interest fused to the C-terminus of Amber | |||
Express a gene of interest fused to the N-terminus of monomeric Venus | |||
Express a gene of interest fused to the C-terminus of monomeric Venus | |||
Expresses mammalian optimized YPet | |||
Expresses YPet with C-terminal His tag | |||
Express a gene of interest fused to the C-terminus of SYFP2 | |||
Expresses Clover (a GFP variant) commonly used with mRuby2 | |||
Express a gene of interest fused to the N-terminus of LSSmOrange | |||
Express a gene of interest fused to the C-terminus of LSSmOrange | |||
Expresses mRuby2 (a RFP variant) commonly used with Clover | |||
Gateway-compatible vector to express a gene of interest fused between ECFP and Venus |
Other issues that can affect FRET measurements include: the brightness of a fluorophore pair, donor:acceptor stoichiometry, and cross-talk between the two fluorophore colours. Experts in FRET recommend to using two fluorophores that have a similar brightness - even though in the most popular pair, CFP has five-fold less brightness than YFP. The stoichiometry of donor:acceptor is difficult to address especially when you investigate unknown molecular complexes. But it is a parameter that you have to keep in mind when analysing FRET results. The cross-talk between the two fluorophores is linked to their excitation spectrum overlap. If you choose a pair too close to each other in the spectrum, you can easily directly excite the acceptor with the laser used to excite the donor. In that case the cross-talk is high and your background signal may be higher than the signal generated by the energy transfer. On the contrary, if you choose a pair that are too far from each other, you don’t have any cross-talk but you also don’t have any resonance transfer. You have to find a balance between FRET efficiency and cross-talk.
Methods to Measure FRET for Cell Biology Studies
Several methods have been used over the past 20 years to measure FRET and there is not one that is better than another. It is often recommended by FRET experts to use as many measurement methods as feasible when first beginning to establish the FRET methodology for a given experiment. You can then choose the most efficient approach for your own system.
The simplest and the most popular one is the sensitised emission method, where the donor is excited by a specific wavelength of light and the signal is collected by using emission filters chosen for the donor fluorescence and the acceptor fluorescence. Additionally, this method could be the best option if there is no cross-talk between FRET pairs. Unfortunately cross-talk between fluorophores does exist in the real world and corrective approaches and appropriate controls are required to make this method useful for dynamic experiments in which FRET changes are large.
Two other methods are commonly used to measure FRET: the acceptor photobleaching method and the fluorescence lifetime imaging microscopy (FLIM) method.
Acceptor photobleaching method
The acceptor photobleaching method is simple but limited to a single measurement. This method is based on the fact that the donor is quenched when FRET occurs. By photobleaching the acceptor, you release the donor's quenching and the fluorescence of the donor is increased. This method is straightforward and quantitative, but it is destructive and cannot be used for dynamic measurements. Extra care should be taken so as not to destroy the donor molecule.
Fluorescence lifetime imaging microscopy method
FLIM has been developed more recently and is the most rigorous method for measuring FRET. FLIM measures the fluorescence decay time of the donor. When FRET occurs between the pairs, donor fluorescence is quenched and the fluorescence decay time of the donor is shortened, allowing FLIM to give an unambiguous value of FRET efficiency. As you don’t measure acceptor fluorescence, this method is also less sensitive to direct acceptor excitation artifacts and it is possible to use a non-fluorescent acceptor. It should be noted that FLIM is a slower imaging method, limiting its use in many FRET experiments. In addition, other environmental factors, such as pH or autofluorescence background, can change the fluorescence decay time and have to be taken into account when interpreting data.
FRET is 20 years old but as you can imagine not old-fashioned at all. With the development of new fluorescent pairs and the emergence of faster measurement systems, this technique has still many good days and good “gigs” ahead...No offense to BRET, CRET and other RET techniques which have never outclassed the Master.
Note:
- BRET= Bioluminescence Resonance Energy Transfer
- CRET= Chemiluminescence Resonance Energy Transfer
Interesting Articles About FRET:
- Piston DW, Kremers GJ. Fluorescent protein FRET: the good, the bad and the ugly. Trends Biochem Sci. 2007 Sep; 32(9): 407-14.
- Zimmermann T, Terjung S. FRET Basics and Applications: An EAMNET Teaching Module http://www.embl.de/eamnet/downloads/modules/FRET_teaching_module.pdf
- Day RN, Davidson MW. Fluorescent proteins for FRET microscopy: monitoring protein interactions in living cells. Bioessays. 2012 May; 34(5):341-50. doi: 10.1002/bies.201100098.
- Sekar RB, Periasamy A. Fluorescence resonance energy transfer (FRET) microscopy imaging of live cell protein localizations. J Cell Biol. 2003 Mar 3;160(5):629-33.
Topics: Fluorescent Proteins, FRET
Leave a Comment