As evidenced by all the CRISPR publications, press, and plasmids out there, it’s obvious that CRISPR is a ground-breaking technology that’s already had a huge impact on research and will be affecting our everyday lives very soon. Not only is CRISPR having effects on various biological disciplines, the base technology itself is constantly improving. Cas9 variants have been modified for genome editing, activating gene expression, visualizing genomic loci, and much more. Now, researchers from the Zhang, Joung, and Doudna labs have improved the on-target specificity of the Cas9 nuclease with engineered variants: eSpCas9, SpCas9-HF1, & HypaCas9.
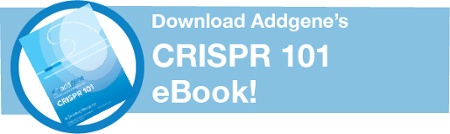
The off-target problem
It is well known that CRISPR/Cas9 genome editing can result in unwanted changes at non-target sites. Means to decrease these so-called “off-target effects” have included using a Cas9 nickase variant, lowering Cas9 expression, and truncating gRNA sequences used for targeting; however, these options can be cumbersome, can lower on-target efficiency, and sometimes even increase off-target effects, respectively. Recognizing these issues, researchers from the Zhang lab at the Broad Institute, the Joung lab at Harvard Medical School, and the Doudna Lab at Berkeley set out to decrease the off target effects of CRISPR/Cas9 by altering the nuclease activity of the Cas9 nuclease itself.
Design and testing of eSpCas9
Looking at the structure of the Cas9 nuclease (PDB ID: 4OO8 and 4UN3), Slaymaker et al. hypothesized that Cas9 cutting efficiency increases when target DNA strand separation is stabilized (2,3). Stable strand separation is maintained by 2 sets of interactions :
1. Interactions between the non-target strand and a positively charged groove formed by the Cas9 HNH and RuvC nuclease domains
2. Interactions between the target strand and the gRNA (see figure below)
Because off-target sequences have less complementarity to the gRNA, they have a higher propensity to reform double helices and decrease Cas9 cutting efficiency. However, this is not always enough to keep off-target sites from being cut. Slaymaker et al reasoned that, if they decreased the positive charge in the HNH/RuvC groove, then they could weaken interactions between the groove and the negatively charged DNA and therefore destabilize basal strand separation. This decreased strand separation combined with the weak separation afforded by gRNA binding at non-target sites would theoretically decrease off-target cutting.
To decrease the electropositivity of the HNH/RuvC groove, Slaymaker et al. made a variety of alanine substitutions throughout the groove in 32 separate Cas9 mutants. When tested for their ability to cut the EMX1 locus in human embryonic kidney (HEK) cells, 11 of these initial mutants retained the on-target activity of wt Cas9 while decreasing off-target activity at known off-target loci. The authors went on to combine the best of these mutations and tested the combination mutants for their on-target and off-target activity at multiple genomic locations in HEK cells. Further testing of two of the mutants, SpCas9(K855A) and eSpCas9(1.1), followed by a technique for detecting genome-wide double strand breaks (BLESS), revealed that these mutants do not cause off-target effects at unanticipated sites and, as predicted, decrease off-target effects genome-wide.
Further work by Slaymaker et al. showed that these mutants are not toxic to HEK cells and that similar mutations can improve the specificity of Cas9 derived from S. aureus. This means you can likely apply similar rational mutations to your Cas9 homolog of choice and get improved on-target specificity.
Design and testing of SpCas9-HF1
Kleinstiver et al and the Joung Lab similarly thought that if they could weaken sequence independent interactions between Cas9 and DNA, then they could diminish off-target cutting (4). However, instead of weakening interaction sbetween the non-target strand and Cas9, Kleinstiver et al disrupted interactions between Cas9 and the phophate backbone of the target DNA strand via mutations N497A, R661A, Q695A, and Q926A (SpCas9-HF1 contains all four mutations). Although the precise mutations were different than those found in eSpCas9, the effect on specificity was similar; SpCas9-HF1 generated fewer off-target cuts when compared to WT SpCas9 across a variety of genomic sites.
Knowledge of Cas9’s dynamic structure leads to the development of HypaCas9
Researchers in the Doudna Lab at Berkeley recently added their own hyper accurate Cas9 variant (HypaCas9) to the pool of enhanced CRISPR tools. In their work, Chen et al. 2017 used single molecule FRET measurements to determine which portions of Cas9 might be important for nuclease specificity. They found that eSpCas9(1.1) and SpCas9-HF1 each stall in an inactive form after binding to a target site and propose that this stalling leads to their enhanced specificity. Additional work revealed that mutations in the mobile Rec3 domain facilitate this stall. With this new found structural knowledge, in collaboration with the Joung Lab, they used targeted mutagenesis to create HypaCas9 (SpCas9 N692A/M694A/Q695A/H698A). This new variant displays comparable on-target activity and similar or decreased off-target activity across the sites tested when compared to eSpCas9(1.1) and SpCas9-HF1.
The HypaCas9 plasmids are available from Addgene here!
Future possibilities for Cas proteins
With their enhanced specificity, eSpCas9, SpCas9-HF1, and HypaCas9 should enable researchers to make precise edits in mammalian cells and may decrease worries about off target effects in applied and/or therapeutic settings. Will combining the different mutations found in each of these great tools further enhance genome editing specificity? We hope one of you will be able to answer this question very soon! We look forward to seeing how the research community makes use of these tools and are excited to see continued improvements in genome engineering!
References
1. Slaymaker, Ian M., et al. "Rationally engineered Cas9 nucleases with improved specificity." Science (2015): aad5227. PubMed PMID: 26628643.
- Find plasmids from this publication at Addgene.
2. Nishimasu, Hiroshi, et al. "Crystal structure of Cas9 in complex with guide RNA and target DNA." Cell 156.5 (2014): 935-949. PubMed PMID: 24529477. PubMed Central PMCID: PMC4139937.
3. Anders, Carolin, et al. "Structural basis of PAM-dependent target DNA recognition by the Cas9 endonuclease." Nature 513.7519 (2014): 569-573. PubMed PMID: 25079318. PubMed Central PMCID: PMC4176945.
4. Kleinstiver, Benjamin P., et al. "High-fidelity CRISPR–Cas9 nucleases with no detectable genome-wide off-target effects." Nature (2016). PubMed PMID: 26735016.
- Find plasmids from this publication at Addgene.
5. Chen, JS, et al. "Enhanced proofreading governs CRISPR-Cas9 targeting accuracy." Nature (2017). PubMed PMID: 28931002.
- Find plasmids from this publication at Addgene.
Resources on the Addgene Blog
- Read about Cas9 Homolog Cpf1
- Read How Non-Homologous End Joining Repairs Double Strand Breaks
- Get Tips on Designing Your gRNA
- Browse Our CRISPR 101 Series
Resources on the Addgene Website
Topics: CRISPR, Cas Proteins
Leave a Comment