Flash quiz! What pops into your head when you hear the phrase “action potential?” Firing neurons are likely the first thing that comes to mind. However, ion-based communication is not just for neurons. Non-neuronal cells do this too. It's just that for non-neural cells, ion-based communication happens at a slightly slower scale.
All living cells with a membrane possess an electrical state. This state, known as the resting potential (Vmem), can be measured as the imbalance of electrical charge between the inside and the outside of the cell. This imbalance is maintained by ion channels and pumps in the membrane. An emerging interdisciplinary field of biology known as bioelectricity is revealing the important role of bioelectrical signaling mediated by ion-channels and pumps in developmental biology, regeneration studies, and cancer biology.
I was first introduced to bioelectricity when I attended the Developmental Bioelectricity satellite symposium at the 2019 Society for Developmental Biology meeting in Boston. The atmosphere was electric! After attending this meeting I had an increased appreciation for the role of ion channels and pumps in developmental biology.
Examples of some ion-channel and ion-pump encoding genes
Examples of some genes encoding gap junction proteins
Bioelectric states can alter cell types and patterning
There is mounting evidence that signaling via ion channels and even between many cells (through gap junction proteins which connect the cytoplasm of two cells) can also affect patterning and in some cases even override genetically encoded patterning. Based upon trends in studies over the past several years, it seems that the more polarized (negative) the action potential of a cell, the more differentiated and quiescent it is, and the more depolarized (positive) the cell is, the more embryonic, stem cell-like, or cancerous it is (Levin et al., 2017). These states can even be necessary and sufficient for certain patterning activities of entire tissue types or even organs. In frogs, ectopic expression of ion channels, despite having an otherwise normal genomic background, can even lead to the formation of whole eyes from cell types that don’t typically give rise to eyes (Pai et al., 2011).
Additionally, researchers have reprogrammed regenerating tissues without using genome engineering. Planarian worms cut into three segments “remember” which side should grow a head and a tail, and when gap junction-mediated electrical communication is briefly inhibited in the entire worm during regeneration, the worm creates two heads. Subsequent cutting results in a two-headed worm, despite the fact that it’s genetically wild type (Oveido et al., 2010).
One of the challenges of studying bioelectricity is that it can be hard to visualize or perturb these slower-changing bioelectric states in living cells. Since bioelectrical signals are controlled by the opening and closing of ion channel proteins, they are not always easy to track at the transcriptional or translational level. However, several genetic tools have been developed to address these hurdles.
Tools for studying bioelectricity
Dyes and other technologies are now available for fluorescent reporting and genetically encoded fluorescent voltage indicators (GEVI) are ideal tools to track bioelectric states (Matthew et al., 2018). These allow for not only recording but also modulating bioelectric states in vivo.
For example, the Levin lab from Tufts University has used Arch (green-light activated proton pump) and ChR2 (blue-light activated cation channel) light-activated channels to hyperpolarize tumor cells, which resulted in tumor regression in a frog model (Chernet et al., 2016). There are many other light-based genetic tools for studying bioelectricity in vivo.
Here are some optogenetic and GEVI tools (as discussed in Mathews and Levin, 2018) available at Addgene:
- MerMAID2 - A metagenomically discovered marine anion-conducting channelrhodopsin used as a sensitive fluorescent voltage indicator with near-complete desensitization in continuous light.
- ArcLight Q239 - A GEVI based on the fusion of the voltage sensing domain of a Ciona intestinalis voltage sensor and a mutated version of the pHLuorin fluorescent protein. This GEVI features increased brightness and improved plasma membrane specificity.
- Stable Step Function Opsin - A stabilized step function opsin (SSFO) for optogenetic activation. Upon activation, exhibits stabilized activity for over 30 minutes and can be deactivated using yellow light (590nm).
- BLINK2 potassium channel - An optimized blue-light induced synthetic potassium channel. Upon activation, it stays open for a longer duration.
- Hyperpolarizing chloride channel SwiChr++ - A channelrhodopsin converted into a chloride-conducting anion channel. It is triggered by a short low-intensity blue light pulse and stays open for a longer duration upon activation.
- p-mcherry-C1-iC++_CA - A slow cycling (open for minutes) step-function artificial anion conducting channelrhodopsin (aACR). It is activated by cyan light and exhibits an inactivation maximum of 590 nm light.
- Aurora and Phobos optogenetic tools - A collection of bi-stable anion optogenetic channels which can be toggled between open and closed states using different wavelength light pulses.
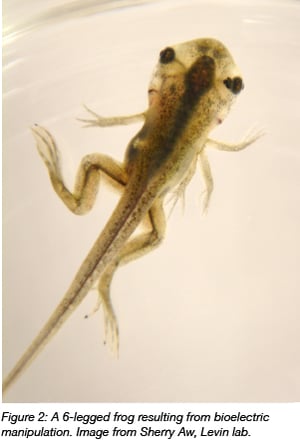
Other resources from the bioelectricity field
In addition to sharing tools to study bioelectricity, bioelectric researchers in many different fields came together to found the journal Bioelectricity in 2019 with a goal to broaden this field of research to the whole scientific community.
Over the years, developmental biologists and cancer researchers are beginning to turn their attention to bioelectricity. One such group, the Allen Discovery Center at Tufts University, studies how bioelectrical signals affect pattern memory and decision-making in somatic cell networks. “Developmental bioelectricity is an extremely exciting emerging field, with many implications for regenerative medicine, evolution of body plans, synthetic morphology, and even artificial intelligence," says Michael Levin, Director of the Allen Discovery Center. The future of this field has a high potential for the advancement of research in birth defects, regeneration, medicine, and synthetic bioengineering.
References
Chernet, Brook T., et al. "Use of genetically encoded, light-gated ion translocators to control tumorigenesis." Oncotarget 7.15 (2016): 19575. PubMed PMID: 26988909. PubMed Central PMCID: PMC4991402.
Levin, Michael, Giovanni Pezzulo, and Joshua M. Finkelstein. "Endogenous bioelectric signaling networks: exploiting voltage gradients for control of growth and form." Annual review of biomedical engineering 19 (2017): 353-387. PubMed PMID: 28633567.
Mathews, Juanita, and Michael Levin. "The body electric 2.0: recent advances in developmental bioelectricity for regenerative and synthetic bioengineering." Current opinion in biotechnology 52 (2018): 134-144. PubMed PMID: 29684787.
Oviedo, Néstor J., et al. "Long-range neural and gap junction protein-mediated cues control polarity during planarian regeneration." Developmental biology 339.1 (2010): 188-199. PubMed PMID: 20026026. PubMed Central PMCID: PMC2823934.
Pai, Vaibhav P., et al. "Transmembrane voltage potential controls embryonic eye patterning in Xenopus laevis." Development 139.2 (2012): 313-323. PubMed PMID: 22159581. PubMed Central PMCID: PMC3243095.
Additional resources on the Addgene blog:
Other resources
- Allen Discovery Center at Tufts
- Developmental Bioelectricity symposium’s “What is Bioelectricity?”
- Journal of Bioelectricity
Topics: Other, Miscellaneous
Leave a Comment