Originally published Jan 27, 2015 and last updated Dec 17, 2020.
This post was contributed by Jordan Ward who is an assistant professor at the University of California, Santa Cruz.
Emerging CRISPR/Cas9 editing technologies have transformed the palette of experiments possible in a wide range of organisms and cell lines. In C. elegans, one of the model organisms which I use to study gene regulation during developmental processes, CRISPR/Cas9 allows us to delete sequences and introduce mutations and epitopes with unprecedented ease. These new C. elegans approaches rapidly enrich for editing events without the need for any selective marker to remain in the edited animal.
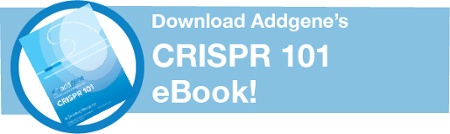
Development of CRISPR/Cas9 editing strategies in C. elegans
As brilliantly and irreverently illustrated in the parable of the Geneticist vs the Biochemist, we geneticists are a lazy bunch who love to rely on the awesome power of genetic selection. Knock-in events tend to be rare, and the challenge with any experiment is in efficient recovery of these edits. Initial work in C. elegans relied on selective markers such as drug resistance (Chen et al., 2013), fluorescence (GFP; Tzur et al., 2013), or rescue of mutant phenotypes (unc-119) (Dickinson et al., 2013). These approaches allowed effective recovery of knock-ins, but did result in 1-2 kilobases of additional sequence being introduced. Cre-mediated excision of the selective cassette minimizes the sequence added, leaving a 34 bp “scar”, but currently requires additional experimental manipulation. In some cases, injection of Cre is required, however the development of the self-excising cassette (Dickinson et al., 2015) made this step as simple as heat-shocking to induce the expression of a Cre recombinase contained within the knock-in. Including Cre-mediated marker excision, it takes approximately 3-4 weeks to obtain a homozygous, outcrossed knock-in ready for experimentation.
In 2014, several manuscripts published in Genetics detailed approaches in which selection for an editing event that produces a visible phenotype enriches for knock-outs and knock-ins at other genomic loci. The first approach – co-CRISPR from Craig Mello’s lab – used inactivation of the unc-22 gene as their selection marker (Kim et al., 2014). Meanwhile Andy Fire’s lab used oligo-mediated knock-in of a dominant mutation, known as co-conversion (Arribere et al., 2014). In both cases, the selected mutation must be removed, which can be done by isolating animals with particular visible phenotypes. These approaches may become even more powerful following a report that one can use linear repair templates (ie. PCR-derived dsDNA) with 30-60 basepair homology arms to knock in large epitopes, such as GFP (Paix et al., 2014). A further development of this approach reported in 2017 uses the direct injection of a CRISPR/Cas9 ribonucleoprotein complex with linear DNA as repair templates which reduces time to generate edits to only four days (Paix et al., 2017). The co-CRISPR and co-conversion approaches have the advantage of being used in any genetic background, but require variable amounts of experimental manipulation and screening.
A co-selection strategy for CRISPR editing in C. elegans improves efficiency
I was fortunate – or unfortunate enough, depending on perspective – to work with inefficient sgRNAs in my initial direct screening efforts, which were similar to the approach detailed by Paix et al. Although I was able to knock-in a 2xFLAG epitope into my gene of interest, I encountered low efficiency (0.13%) and laborious handling (screening 768 F1 animals). Recovering rare editing events in a sea of unedited animals struck me as a “needle in the haystack” type of problem and led to me to explore alternate approaches. Being a “lazy” geneticist, I developed a co-selection approach relying on repair of a conditional-lethal mutation to identify edits with minimal screening effort. Selecting for repair of a lethal mutation should remove much of the unedited “haystack”, facilitating recovery of edited animals.
![]() |
Figure 1: In the background are 504 restriction digests of PCR products used to identify a single 2xFLAG knock-in by direct screening. The center images depict the power of lethal mutation co-selection. Only animals rescued for the conditional lethal mutation survive the selection; from a representative co-selection experiment, five knock-ins were recovered from seven rescued F1 animals (gel image under worm). Image courtesy of Jordan Ward, UCSF. |
In 2015 (Ward, 2015), I demonstrated that selection for repair of a temperature-sensitive pha-1 mutation significantly enriches for knock-in of 2x and 3xFLAG epitopes into other, non-linked loci; pha-1(e2123) mutant worms are perfectly viable at 15 ºC, yet exhibit complete embryonic lethality at 25 ºC. This method resulted in efficiencies ranging from 11-100% of F1 animals carrying precise knock-ins, and homozygous knock-in animals can be obtained in eight days. The only animals on a plate, other than the parental animal, are rescued progeny, which makes screening extremely rapid. This stringent selection allowed me to optimize a range of editing parameters: oligo repair templates with homology arms of 35-80 bp, and DNA double-strand breaks up to 54 bp from the desired insertion site result in efficient editing. Repair oligos do not need to be PAGE purified, although doing so increases knock-in efficiency. Finally, as shown in Drosophila S2 cells (Böttcher et al., 2014), inactivation of non-homologous end-joining results in a further increase in knock-in efficiency, presumably by channeling DNA breaks into the homologous recombination repair pathway. Reagents required to perform pha-1 co-conversion are available through Addgene. In 2019, Farboud et al. described another similar co-conversion marker (repair of a zen-4(cle10ts) allele), increasing experimental options.
Expanding and improving the CRISPR/Cas editing toolbox for C. elegans
Recent work has further improved the efficiency of genome editing by CRISPR whether it be by improved gRNA or repair template design, delivery, screening, and more. Here are some highlights from the past few years:
Repair template design
The Meyer lab meticulously characterized several aspects of editing. First, they devised guidelines for designing single-stranded repair templates (Farboud et al., 2019).
The Mello lab also reported that modifying the 5’ modification of oligos used to generate linear dsDNA repair templates by PCR boosted efficiency (Ghanta et al., 2018). They further built on this work, discovering that melting and rapidly cooling dsDNA repair templates dramatically boosted editing efficiency (Ghanta et al., 2020). They also found that the majority of knock-ins were occurring 24 hours post-injection, making screening for knock-ins much more efficient. Pure PCR repair templates are critical for efficient knock-ins (Ghanta et al., 2020). Together, these improvements from the Mello lab resulted in strikingly high editing efficiencies, obtaining as many as 100 independent GFP knock-ins from a single injected animal.
PAM sequence considerations
For changes 5’ to the PAM, sequences corresponding to the same strand as the PAM produced a higher editing efficiency, while for edits 3’ to the PAM sequences corresponding to the opposite strand were better (Farboud et al., 2019).
The Meyer lab also determined optimal PAM orientation for editing using two Cas9 targets. An orientation where the 5’ ends of the PAMs are facing outwards, ensuring the PAMs remain on the chromosome after DSB formation, was most efficient (Farboud et al., 2019). Using this approach, they were able to insert 10 kb of non-homologous sequence or a series of single-nucleotide substitutions along a 1.5 kb tract, all without selectable markers.
CRISPR in C. elegans
The Meyer and Mello labs also identified several experimental set ups that could improve CRISPR efficiency in C. elegans. The Meyer lab also found that mating improved editing efficiency suggesting that sperm are more permissive to Cas9 editing (Farboud et al., 2019). Work from the Mello lab has dramatically improved RNP editing with linear repair templates. They found that Cas9 RNPs could be toxic at high concentrations, and editing efficiency improved by using more dilute mixtures (Doksin et al., 2018). The Mello lab also reported efficient editing using a co-injection marker that is mainly lost in F2 animals, removing the need to segregate away co-conversion markers.
Expanding beyond Cas9
Since the development of CRISPR/Cas9 editing methods, other Cas proteins have been adapted for use in C. elegans. In 2017, the Korswagen lab developed Cas12a (Cpf1) for editing in C. elegans (Ebbing et al., 2017). Because C. elegans has an AT-rich genome, it can be difficult to find an appropriate NGG PAM site to target. The (T)TTN PAM motif required by the Acidaminococcus sp. BV3L6 Cas12a (AsCpf1) eases this constraint and allows editing from more sites of the C. elegans genome.
Toolkits for editing in C. elegans
The development of toolkits such as the SapTrap CRISPR/Cas Toolkit and SapTrap-SEC kit for C. elegans genome engineering have also facilitated CRISPR editing in C. elegans. These modular toolkits build single plasmid targeting vectors that encode the gRNA transcript and the repair template of your choosing. Tools such as SapTrap Builder greatly simplifies design of these constructs, allowing novice researchers to quickly and easily start genome editing (Schwartz et al., 2018).
To see more CRISPR tools for C. elegans, visit Addgene’s C. elegans CRISPR plasmids page.
Future perspectives
It is interesting to note that recipients for the 2015 Breakthrough Prize included Jennifer Doudna and Emmanuelle Charpentier for their pioneering CRISPR/Cas9 work (now recognized by the 2020 Nobel Prize in Chemistry), and Victor Ambros and Gary Ruvkun for their seminal work on micro RNAs in C. elegans. The C. elegans work on small RNAs informed and drove work in countless other systems, whereas import of CRISPR technology into C. elegans has been a transformative innovation for our community. Developments in mammalian and yeast cells using modified Cas9 proteins to regulate gene expression (CRISPRi/CRISPRa), visualize specific genomic loci (CRISPR-imaging), or to find the proteins associated with a given genomic locus (CRISPR-ChAP-MS) could further transform the range of experiments imaginable in C. elegans. Equally, some of the “elegans” methods outlined in this post – particularly the use of co-selection methods – could greatly streamline editing in other organisms and systems, allowing rapid progress in a wide range of fields.
Thank you to our guest blogger!
Jordan Ward is an assistant professor at the University of California, Santa Cruz where his group focuses on gene regulation in nematode molting and spermatogenesis. His group also builds tools to improve genome editing and conditionally manipulate proteins. Learn more at his webpage or follow him on Twitter.
References
Arribere JA, Bell RT, Fu BXH, Artiles KL, Hartman PS, Fire AZ (2014) Efficient Marker-Free Recovery of Custom Genetic Modifications with CRISPR/Cas9 in Caenorhabditis elegans. Genetics 198:837–846 . https://doi.org/10.1534/genetics.114.169730
Bottcher R, Hollmann M, Merk K, Nitschko V, Obermaier C, Philippou-Massier J, Wieland I, Gaul U, Forstemann K (2014) Efficient chromosomal gene modification with CRISPR/cas9 and PCR-based homologous recombination donors in cultured Drosophila cells. Nucleic Acids Research 42:e89–e89 . https://doi.org/10.1093/nar/gku289
Chen C, Fenk LA, de Bono M (2013) Efficient genome editing in Caenorhabditis elegans by CRISPR-targeted homologous recombination. Nucleic Acids Research 41:e193–e193 . https://doi.org/10.1093/nar/gkt805
Dickinson DJ, Pani AM, Heppert JK, Higgins CD, Goldstein B (2015) Streamlined Genome Engineering with a Self-Excising Drug Selection Cassette. Genetics 200:1035–1049 . https://doi.org/10.1534/genetics.115.178335
Dokshin GA, Ghanta KS, Piscopo KM, Mello CC (2018) Robust Genome Editing with Short Single-Stranded and Long, Partially Single-Stranded DNA Donors in Caenorhabditis elegans. Genetics 210:781–787 . https://doi.org/10.1534/genetics.118.301532
Ebbing A, Shang P, Geijsen N, Korswagen HC (2017) Extending the CRISPR toolbox for C. elegans: Cpf1 as an alternative gene editing system for AT-rich sequences https://doi.org/10.17912/W2237D
Farboud B, Severson AF, Meyer BJ (2018) Strategies for Efficient Genome Editing Using CRISPR-Cas9. Genetics 211:431–457 . https://doi.org/10.1534/genetics.118.301775
Ghanta KS, Doksin GA, Mir A, Krishnamurthy PM, Gneid H, Edraki A, Watts JK, Sontheimer EJ, Mello CC (2018) 5′ Modifications Improve Potency and Efficacy of DNA Donors for Precision Genome Editing. BioRxiv. https://doi.org/10.1101/354480
Ghanta KS, Mello CC (2020) Melting dsDNA Donor Molecules Greatly Improves Precision Genome Editing in Caenorhabditis elegans. Genetics 216:643–650 . https://doi.org/10.1534/genetics.120.303564
Kim H, Ishidate T, Ghanta KS, Seth M, Conte D Jr, Shirayama M, Mello CC (2014) A Co-CRISPR Strategy for Efficient Genome Editing inCaenorhabditis elegans. Genetics 197:1069–1080 . https://doi.org/10.1534/genetics.114.166389
Paix A, Folkmann A, Seydoux G (2017) Precision genome editing using CRISPR-Cas9 and linear repair templates in C. elegans. Methods 121–122:86–93 . https://doi.org/10.1016/j.ymeth.2017.03.023
Paix A, Wang Y, Smith HE, Lee C-YS, Calidas D, Lu T, Smith J, Schmidt H, Krause MW, Seydoux G (2014) Scalable and Versatile Genome Editing Using Linear DNAs with Microhomology to Cas9 Sites in Caenorhabditis elegans. Genetics 198:1347–1356 . https://doi.org/10.1534/genetics.114.170423
Schwartz ML, Jorgensen EM (2018) SapTrap vectors for introducing point mutations with unc-119+ selection. https://doi.org/10.17912/DDVH-BG64
Tzur YB, Friedland AE, Nadarajan S, Church GM, Calarco JA, Colaiácovo MP (2013) Heritable Custom Genomic Modifications inCaenorhabditis elegansvia a CRISPR–Cas9 System. Genetics 195:1181–1185 . https://doi.org/10.1534/genetics.113.156075
Ward JD (2014) Rapid and Precise Engineering of theCaenorhabditis elegansGenome with Lethal Mutation Co-Conversion and Inactivation of NHEJ Repair. Genetics 199:363–377 . https://doi.org/10.1534/genetics.114.172361
Want to learn more about genome editing with CRISPRs?
- Check-out Addgene's CRISPR Resources
- Learn about gRNA design and gRNA optimization for your CRISPR experiment
Topics: CRISPR, Other CRISPR Tools, Worm
Leave a Comment